Thermodynamics
Table of Contents
Energy can be transferred to or from a system, either through a temperature difference between it andanother system (i.e., by heat) or by exerting a force through a distance (work). In these ways, energy can be converted into otherforms of energy in other systems. For example, a car engine burns fuel for heat transfer into a gas. Work is done by the gas as it exerts a force through a distance by pushing a piston outward. This work converts the energy into a variety of other forms—intoan increase in the car’s kinetic or gravitational potential energy; into electrical energy to run the spark plugs, radio, and lights;and back into stored energy in the car’s battery. But most of the thermal energy transferred by heat from the fuel burning in theengine does not do work on the gas. Instead, much of this energy is released into the surroundings at lower temperature (i.e.,lost through heat), which is quite inefficient. Car engines are only about 25 to 30 percent efficient. This inefficiency leads toincreased fuel costs, so there is great interest in improving fuel efficiency. However, it is common knowledge that moderngasoline engines cannot be made much more efficient. The same is true about the conversion to electrical energy in large powerstations, whether they are coal, oil, natural gas, or nuclear powered. Why is this the case?
The answer lies in the nature of heat. Basic physical laws govern how heat transfer for doing work takes place and limit themaximum possible efficiency of the process. This chapter will explore these laws as well their applications to everyday machines.
This chapter will explore these laws as well their applications to everyday machines. These topics are part of thermodynamics—the study of heat and its relationship to doing work.
Zeroth Law of Thermodynamics: Thermal Equilibrium
When two objects (or systems) are in contact with one another, heat will transfer thermal energy from the object at higher temperature to the one at lower temperature until they both reach the same temperature. The objects are then in thermal equilibrium, and no further temperature changes will occur if they are isolated from other systems. The systems interact and change because their temperatures are different, and the changes stop once their temperatures are the same. Thermal equilibrium is established when two bodies are in thermal contact with each other—meaning heat transfer (i.e., the transfer of energy by heat) can occur between them. If two systems cannot freely exchange energy, they will not reach thermal equilibrium. (It is fortunate that empty space stands between Earth and the sun, because a state of thermal equilibrium with the sun would be too toasty for life on this planet!)
If two systems, A and B, are in thermal equilibrium with each another, and B is in thermal equilibrium with a third system, C, then A is also in thermal equilibrium with C. This statement may seem obvious, because all three have the same temperature, but it is basic to thermodynamics. It is called the zeroth law of thermodynamics.
The zeroth law of thermodynamics is very similar to the transitive property of equality in mathematics: If a = b and b = c, then a = c
Pressure, Volume, Temperature, and the Ideal Gas Law
Before covering the first law of thermodynamics, it is first important to understand the relationship between pressure, volume, and temperature. Pressure, P, is defined as
P = F / A
where F is a force applied to an area, A, that is perpendicular to the force. Depending on the area over which it is exerted, a given force can have a significantly different effect
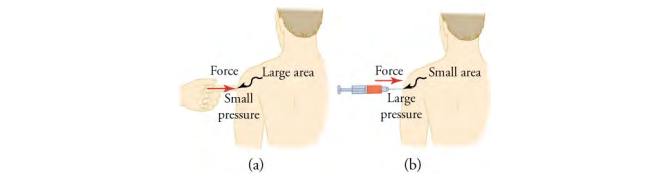
The SI unit for pressure is the pascal, where Pressure is defined for all states of matter but is particularly important when discussing fluids (such as air).
You have probably heard the word pressure being used in relation to blood (high or low blood pressure) and in relation to the weather (high- and low-pressure weather systems). These are only two of many examples of pressures in fluids.
The relationship between the pressure, volume, and temperature for an ideal gas is given by the ideal gas law. A gas is considered ideal at low pressure and fairly high temperature, and forces between its component particles can be ignored.
The ideal gas law states that
PV = NkT
where P is the pressure of a gas, V is the volume it occupies, N is the number of particles (atoms or molecules) in the gas, , and T is its absolute temperature. The constant k is called the Boltzmann constant and has the value ( k = 1.38 X 10-23 J/K)
It is important for us to notice from the equation that the following are true for a given mass of gas:
• When volume is constant, pressure is directly proportional to temperature.
• When temperature is constant, pressure is inversely proportional to volume.
• When pressure is constant, volume is directly proportional to temperature.
This last point describes thermal expansion—the change in size or volume of a given mass with temperature. What is the underlying cause of thermal expansion? An increase in temperature means that there’s an increase in the kinetic energy of the individual atoms. Gases are especially affected by thermal expansion, although liquids expand to a lesser extent with similar increases in temperature, and even solids have minor expansions at higher temperatures. This is why railroad tracks and bridges have expansion joints that allow them to freely expand and contract with temperature changes.
To get some idea of how pressure, temperature, and volume of a gas are related to one another, consider what happens when you pump air into a deflated tire. The tire’s volume first increases in direct proportion to the amount of air injected, without much increase in the tire pressure. Once the tire has expanded to nearly its full size, the walls limit volume expansion. If you continue to pump air into tire (which now has a nearly constant volume), the pressure increases with increasing temperature)
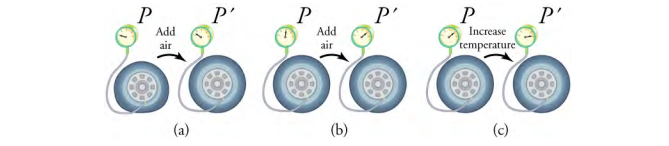
(a) When air is pumped into a deflated tire, its volume first increases without much increase in pressure.
(b) When the tire is filled to a certain point, the tire walls resist further expansion, and the pressure increases as more air is added. (
c) Once the tire is inflated fully, its pressure increases with temperature.
The First Law of Thermodynamics :
Heat (Q) and work (W) are the two ways to add or remove energy from a system. The processes are very different. Heat is driven by temperature differences, while work involves a force exerted through a distance. Nevertheless, heat and work can produce identical results. For example, both can cause a temperature increase. Heat transfers energy into a system, such as when the sun warms the air in a bicycle tire and increases the air’s temperature. Similarly, work can be done on the system, as when the bicyclist pumps air into the tire. Once the temperature increase has occurred, it is impossible to tell whether it was caused by heat or work. Heat and work are both energy in transit—neither is stored as such in a system. However, both can change the internal energy, U, of a system.
Internal energy is the sum of the kinetic and potential energies of a system’s atoms and molecules. It can be divided into many subcategories, such as thermal and chemical energy, and depends only on the state of a system (that is, P, V, and T), not on how the energy enters or leaves the system.
In order to understand the relationship between heat, work, and internal energy, we use the first law of thermodynamics. The first law of thermodynamics applies the conservation of energy principle to systems where heat and work are the methods of transferring energy into and out of the systems. It can also be used to describe how energy transferred by heat is converted and transferred again by work.
The first law of thermodynamics states that the change in internal energy of a closed system equals the net heat transfer into the system minus the net work done by the system. In equation form, the first law of thermodynamics is
ΔU = Q – W
Here, ΔU is the change in internal energy, U, of the system. As shown in Figure 12.6, Q is the net heat transferred into the system—that is, Q is the sum of all heat transfers into and out of the system. W is the net work done by the system—that is, W is the sum of all work done on or by the system. By convention, if Q is positive, then there is a net heat transfer into the system; if W is positive, then there is net work done by the system. So positive Q adds energy to the system by heat, and positive W takes energy from the system by work. Note that if heat transfers more energy into the system than that which is done by work, the difference is stored as internal energy

The first law of thermodynamics is the conservation of energy principle stated for a system, where heat and work are the methods of transferring energy to and from a system. Q represents the net heat transfer—it is the sum of all transfers of energy by heat into and out of the system. Q is positive for net heat transfer into the system. Wout is the work done by the system, and Win is the work done on the system. W is the total work done on or by the system. W is positive when more work is done by the system than on it. The change in the internal energy of the system, ΔU , is related to heat and work by the first law of thermodynamics: ΔU = Q – W
It follows also that negative Q indicates that energy is transferred away from the system by heat and so decreases the system’s internal energy, whereas negative W is work done on the system, which increases the internal energy.
Second Law of Thermodynamics: Entropy
It is not even theoretically possible for engines to be 100 percent efficient. This phenomenon is explained by the second law of thermodynamics, which relies on a concept known as entropy. Entropy is a measure of the disorder of a system. Entropy also describes how much energy is not available to do work. The more disordered a system and higher the entropy, the less of a system’s energy is available to do work.
Although all forms of energy can be used to do work, it is not possible to use the entire available energy for work. Consequently, not all energy transferred by heat can be converted into work, and some of it is lost in the form of waste heat—that is, heat that does not go toward doing work. The unavailability of energy is important in thermodynamics; in fact, the field originated from efforts to convert heat to work, as is done by engines.
The equation for the change in entropy, ΔS , is ΔS = Q/T
where Q is the heat that transfers energy during a process, and T is the absolute temperature at which the process takes place. Q is positive for energy transferred into the system by heat and negative for energy transferred out of the system by heat. In SI, entropy is expressed in units of joules per kelvin (J/K). If temperature changes during the process, then it is usually a good approximation (for small changes in temperature) to take T to be the average temperature
The second law of thermodynamics states that the total entropy of a system either increases or remains constant in any spontaneous process; it never decreases.
An important implication of this law is that heat transfers energy spontaneously from higher- to lower-temperature objects, but never spontaneously in the reverse direction. This is because entropy increases for heat transfer of energy from hot to cold. Because the change in entropy is Q/T, there is a larger change in at lower temperatures (smaller T). The decrease in entropy of the hot (larger T) object is therefore less than the increase in entropy of the cold (smaller T) object, producing an overall increase in entropy for the system
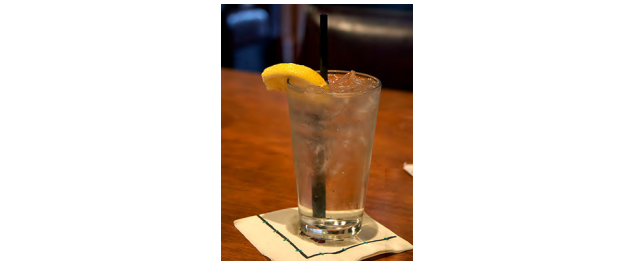
The ice in this drink is slowly melting. Eventually, the components of the liquid will reach thermal equilibrium, as predicted by the second law of thermodynamics—that is, after heat transfers energy from the warmer liquid to the colder ice
Another way of thinking about this is that it is impossible for any process to have, as its sole result, heat transferring energy from a cooler to a hotter object. Heat cannot transfer energy spontaneously from colder to hotter, because the entropy of the overall system would decrease.
Suppose we mix equal masses of water that are originally at two different temperatures, say and . The result will be water at an intermediate temperature of 300 C . Three outcomes have resulted: entropy has increased, some energy has become unavailable to do work, and the system has become less orderly.
Let us think about each of these results. First, why has entropy increased? Mixing the two bodies of water has the same effect as the heat transfer of energy from the higher-temperature substance to the lower-temperature substance. The mixing decreases the entropy of the hotter water but increases the entropy of the colder water by a greater amount, producing an overall increase in entropy.
Second, once the two masses of water are mixed, there is no more temperature difference left to drive energy transfer by heat and therefore to do work. The energy is still in the water, but it is now unavailable to do work.
Third, the mixture is less orderly, or to use another term, less structured. Rather than having two masses at different temperatures and with different distributions of molecular speeds, we now have a single mass with a broad distribution of molecular speeds, the average of which yields an intermediate temperature. These three results—entropy, unavailability of energy, and disorder—not only are related but are, in fact, essentially equivalent. Heat transfer of energy from hot to cold is related to the tendency in nature for systems to become disordered and for less energy to be available for use as work.
Based on this law, what cannot happen? A cold object in contact with a hot one never spontaneously transfers energy by heat to the hot object, getting colder while the hot object gets hotter. Nor does a hot, stationary automobile ever spontaneously cool off and start moving
We’ve explained that heat never transfers energy spontaneously from a colder to a hotter object. The key word here is spontaneously. If we do work on a system, it is possible to transfer energy by heat from a colder to hotter object. We’ll learn more about this in the next section, covering refrigerators as one of the applications of the laws of thermodynamics. Sometimes people misunderstand the second law of thermodynamics, thinking that based on this law, it is impossible for entropy to decrease at any particular location. But, it actually is possible for the entropy of one part of the universe to decrease, as long as the total change in entropy of the universe increases. In equation form, we can write this as
ΔStot = ΔSsystem + ΔSenvironment > 0
Based on this equation, we see that ΔSsystem can be negative as long as ΔSenvironment is positive and greater in magnitude.
How is it possible for the entropy of a system to decrease? Energy transfer is necessary.
- If you pick up marbles that are scattered about the room and put them into a cup, your work has decreased the entropy of that system.
- If you gather iron ore from the ground and convert it into steel and build a bridge, your work has decreased the entropy of that system. . In this case , although you made the system of the bridge and steel more structured, you did so at the expense of the universe. Altogether, the entropy of the universe is increased by the disorder created by digging up the ore and converting it to stee
- Energy coming from the sun can decrease the entropy of local systems on Earth—that is, is negative. But the overall entropy of the rest of the universe increases by a greater amount—that is, is positive and greater in magnitude
Take Quiz
1. What is the net heat out of the system when 25 J is transferred by heat into the system and 45 J is transferred out of it ?
a) -70 J
b) – 20 J
c) 20 J
d) 70 J
ANSWER
c) 20 J
2) What is internal energy?
a. It is the sum of the kinetic energies of a system’s atoms and molecules.
b. It is the sum of the potential energies of a system’s atoms and molecules.
c. It is the sum of the kinetic and potential energies of a system’s atoms and molecules.
d. It is the difference between the magnitudes of the kinetic and potential energies of a system’s atoms and molecules.
ANSWER
c) It is the sum of the kinetic and potential energies of a system’s atoms and molecules.
3) What is the increase in entropy when 3 Kg of ice at 0 0 celsius melt to form water at 0 0 degree celsius ?
a . 1.84 X 10 3 J / K
b. 3.67 X 10 3 J / K
c. 1.84 X 10 8 J / K
d. 3.67 X 10 8 J / K
ANSWER
b) 3.67 X 10 3 J / K
4) For heat transferring energy from a high to a low temperature, what usually happens to the entropy of the whole system?
a. It decreases.
b. It must remain constant.
c. The entropy of the system cannot be predicted without specific values for the temperatures.
d. It increases.
ANSWER
d) It increases.
5) A heat engine is given 120 J by heat and releases 20 J by heat to the environment. What is the amount of work done by the system?
a. – 100 J
b. -60 J
c. 60 J
d. 100 J
ANSWER
d) 100 J
6) Eighty joules are added by heat to a system, while it does 20 J of work. Later 30 J, are added by heat to the system, and it does 40 J of work. What is the change in the system’s internal energy ?
a. 30 J
b. 60 J
c. 50 J
d. 110 J
ANSWER
c) 50 J
7) A coal power station functions at 40.0 percent efficiency. What is the amount of work it does if it takes in 1.20 ×10 12 J by heat?
a. 3 X 1010 J
b. 4.8 X 1011 J
c. 3 X 1012 J
d. 4.8 X 1018 J
ANSWER
b) 4.8 X 1011 J
8) When does a real gas behave like an ideal gas ?
a. A real gas behaves like an ideal gas at high temperature and low pressure.
b. A real gas behaves like an ideal gas at high temperature and high pressure.
c. A real gas behaves like an ideal gas at low temperature and low pressure.
d. A real gas behaves like an ideal gas at low temperature and high pressure.
ANSWER
a) A real gas behaves like an ideal gas at high temperature and low pressure.
9) If Q is 120 J and T is 350 K , what is the change in entropy?
a. 1.51 J / K
b. 0.343 J / K
c.2.92 J / K
d.34.3 J / K
ANSWER
b) 0.343 J / K
10) 310 joules of heat enter a system, after which the system does 120 J of work. What is the change in its internal energy? Would this amount change if 30 J of energy ransferred by heat were added after the work was done instead of before?
a. – 190 J ; this would change if heat added energyafter the work was done
b. 190 J ; this would change if heat added energy after the work was done
c. ; -190 Jthis would not change even if heat added energy after the work was done
d. 190 J ; this would not change even if heat added energy after the work was done
ANSWER
b) 190 J ; this would change if heat added energy after the work was done
11) Why does entropy increase when ice melts into water?
a. Melting converts the highly ordered solid structure into a disorderly liquid, thereby increasing entropy.
b. Melting converts the highly ordered liquid into a disorderly solid structure, thereby increasing entropy.
c. Melting converts the highly ordered solid structure into a disorderly solid structure, thereby increasing entropy.
d. Melting converts the highly ordered liquid into a disorderly liquid, thereby increasing entropy.
ANSWER
a) Melting converts the highly ordered solid structure into a disorderly liquid, thereby increasing entropy.
12) Two distinct systems have the same amount of stored internal energy. 500 joules are added by heat to the first system, and 300 J are added by heat to the second system. What will be the change in internal energy of the first system if it does 200 J of work? How much work will the second system have to do in order to have the same internal energy?
a. 700 J; 0 J
b. 300 J; 300 J
c. 700 J; 300 J
d. 300 J; 0 J
ANSWER
d) 300 J; 0 J
13) A coal power station transfers 3.0×1012 J by heat from burning coal and transfers 1.5×1012 J by heat into the environment. What is the efficiency of the power station?
a.0.33
b. 0.5
c. 0.66
d.1
ANSWER
b) 0.5
14) By convention, if Q is positive, what is the direction in which heat transfers energy with regard to the system?
a. The direction of the heat transfer of energy depends on the changes in W, regardless of the sign of Q.
b. The direction of Q cannot be determined from just the sign of Q.
c. The direction of net heat transfer of energy will be out of the system.
d. The direction of net heat transfer of energy will be into the system
ANSWER
d) The direction of net heat transfer of energy will be into the system
15) What is the change in entropy caused by melting 5.00 kg of ice at 0 °C ?
a.0 J / K
b. 6.11 X 10 3 J / K
c. 6.11 X 10 4 J / K
d. Infinite
ANSWER
b) 6.11 X 10 3 J / K