LAWS OF MOTION
Table of Contents
Motion itself can be beautiful, such as a dolphin jumping out of the water, the flight of a bird, or the orbit of a satellite. The study of motion is called kinematics, but kinematics describes only the way objects move—their velocity and their acceleration. Dynamics considers the forces that affect the motion of moving objects and systems.
Newton’s laws of motion are the foundation of dynamics. These laws describe the way objects speed up, slow down, stay in motion, and interact with other objects. They are also universal laws: they apply everywhere on Earth as well as in space. A force pushes or pulls an object. The object being moved by a force could be an inanimate object, a table, or an animate object, a person. The pushing or pulling may be done by a person, or even the gravitational pull of Earth. Forces have different magnitudes and directions; this means that some forces are stronger than others and can act in different directions.
For example, a cannon exerts a strong force on the cannonball that is launched into the air. In contrast, a mosquito landing on your arm exerts only a small force on your arm. When multiple forces act on an object, the forces combine. Adding together all of the forces acting on an object gives the total force, or net force.
An external force is a force that acts on an object within the system from outside the system. This type of force is different than an internal force, which acts between two objects that are both within the system. The net external force combines these two definitions; it is the total combined external force.
We discuss further details about net force, external force, and net external force in the coming sections. In mathematical terms, two forces acting in opposite directions have opposite signs (positive or negative). By convention, the negative sign is assigned to any movement to the left or downward. If two forces pushing in opposite directions are added together, the larger force will be somewhat cancelled out by the smaller force pushing in the opposite direction. It is important to be consistent with your chosen coordinate system within a problem; for example, if negative values are assigned to the downward direction for velocity, then distance, force, and acceleration should also be designated as being negative in the downward direction.
Newton’s First Law and Friction :
Newton’s first law of motion states the following:
1. A body at rest tends to remain at rest.
2. A body in motion tends to remain in motion at a constant velocity unless acted on by a net external force. (Recall that constant velocity means that the body moves in a straight line and at a constant speed.)
At first glance, this law may seem to contradict your everyday experience. You have probably noticed that a moving object will usually slow down and stop unless some effort is made to keep it moving. The key to understanding why, for example, a sliding box slows down (seemingly on its own) is to first understand that a net external force acts on the box to make the box slow down. Without this net external force, the box would continue to slide at a constant velocity (as stated in Newton’s first law of motion). What force acts on the box to slow it down? This force is called friction.
Friction is an external force that acts opposite to the direction of motion (see Figure ). Think of friction as a resistance to motion that slows things down.
Consider an air hockey table. When the air is turned off, the puck slides only a short distance before friction slows it to a stop. However, when the air is turned on, it lifts the puck slightly, so the puck experiences very little friction as it moves over the surface. With friction almost eliminated, the puck glides along with very little change in speed. On a frictionless surface, the puck would experience no net external force (ignoring air resistance, which is also a form of friction). Additionally, if we know enough about friction, we can accurately predict how quickly objects will slow down.
Now let’s think about an example. A man pushes a box across a floor at constant velocity by applying a force of +50 N. (The positive sign indicates that, by convention, the direction of motion is to the right.) What is the force of friction that opposes the motion? The force of friction must be −50 N. Why? According to Newton’s first law of motion, any object moving at constant velocity has no net external force acting upon it, which means that the sum of the forces acting on the object F net must be zero. The mathematical way to say that no net external force acts on an object is F net = 0 or ∑ F = 0 So if the man applies +50 N of force, then the force of friction must be −50 N for the two forces to add up to zero (that is, for the two forces to cancel each other). Whenever you encounter the phrase “at constant velocity” , Newton’s first law tells you that the net external force is zero
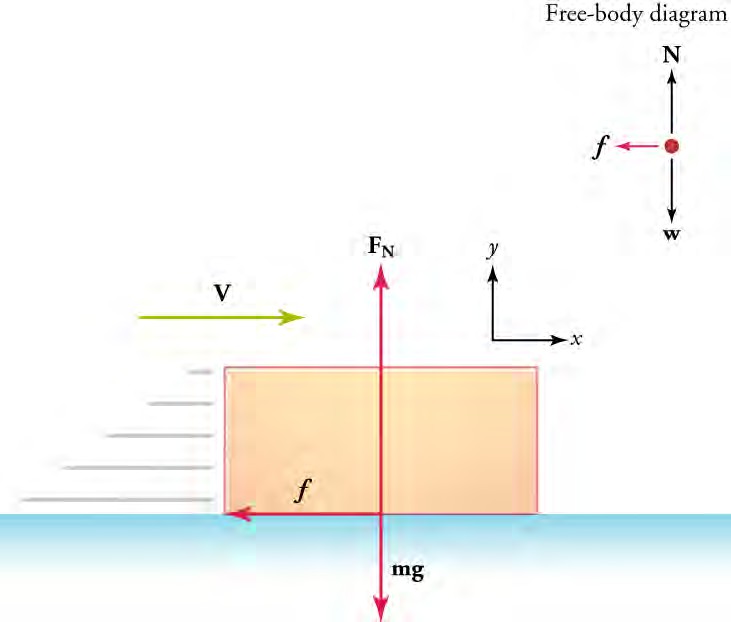
The force of friction depends on two factors: the coefficient of friction and the normal force. For any two surfaces that are in contact with one another, the coefficient of friction is a constant that depends on the nature of the surfaces. The normal force is the force exerted by a surface that pushes on an object in response to gravity pulling the object down. In equation form, the force of friction is
f = µ N
Where µ is the coefficient of friction and N is the normal force.
A net external force acts from outside on the object of interest. A more precise definition is that it acts on the system of interest. A system is one or more objects that you choose to study. It is important to define the system at the beginning of a problem to figure out which forces are external and need to be considered, and which are internal and can be ignored.
For example, in the below Figure , two children push a third child in a wagon at a constant velocity. The system of interest is the wagon plus the small child, as shown in part (b) of the figure. The two children behind the wagon exert external forces on this system (F1, F2). Friction f acting at the axles of the wheels and at the surface where the wheels touch the ground two other external forces acting on the system. Two more external forces act on the system: the weight W of the system pulling down and the normal force N of the ground pushing up. Notice that the wagon is not accelerating vertically, so Newton’s first law tells us that the normal force balances the weight. Because the wagon is moving forward at a constant velocity, the force of friction must have the same strength as the sum of the forces applied by the two children
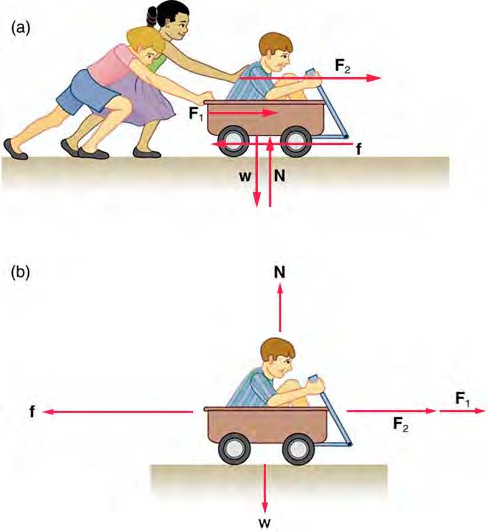
Figure The wagon and rider form a system that is acted on by external forces. (b) The two children pushing the wagon and child provide two external forces. Friction acting at the wheel axles and on the surface of the tires where they touch the ground provide an external force that act against the direction of motion. The weight W and the normal force N from the ground are two more external forces acting on the system. All external forces are represented in the figure by arrows. All of the external forces acting on the system add together, but because the wagon moves at a constant velocity, all of the forces must add up to zero
Mass and Inertia
Inertia is the tendency for an object at rest to remain at rest, or for a moving object to remain in motion in a straight line with constant speed. This key property of objects was first described by Galileo. Later, Newton incorporated the concept of inertia into his first law, which is often referred to as the law of inertia. As we know from experience, some objects have more inertia than others. For example, changing the motion of a large truck is more difficult than changing the motion of a toy truck. In fact, the inertia of an object is proportional to the mass of the object. Mass is a measure of the amount of matter (or stuff) in an object. The quantity or amount of matter in an object is determined by the number and types of atoms the object contains. Unlike weight (which changes if the gravitational force changes), mass does not depend on gravity. The mass of an object is the same on Earth, in orbit, or on the surface of the moon. In practice, it is very difficult to count and identify all of the atoms and molecules in an object, so mass is usually not determined this way. Instead, the mass of an object is determined by comparing it with the standard kilogram. Mass is therefore expressed in kilograms.
Newton’s Second Law of Motion :
Newton’s first law considered bodies at rest or bodies in motion at a constant velocity. The other state of motion to consider is when an object is moving with a changing velocity, which means a change in the speed and/or the direction of motion. This type of motion is addressed by Newton’s second law of motion, which states how force causes changes in motion. Newton’s second law of motion is used to calculate what happens in situations involving forces and motion, and it shows the mathematical relationship between force, mass, and acceleration. Mathematically, the second law is most often written as
Fnet = m a or ∑ F = m a
where Fnet (or ∑F) is the net external force, m is the mass of the system, and a is the acceleration. Note that Fnet and ∑F are the same because the net external force is the sum of all the external forces acting on the system.
what do we mean by a change in motion? A change in motion is simply a change in velocity: the speed of an object can become slower or faster, the direction in which the object is moving can change, or both of these variables may change. A change in velocity means, by definition, that an acceleration has occurred.
Newton’s first law says that only a nonzero net external force can cause a change in motion, so a net external force must cause an acceleration. Note that acceleration can refer to slowing down or to speeding up. Acceleration can also refer to a change in the direction of motion with no change in speed, because acceleration is the change in velocity divided by the time it takes for that change to occur, and velocity is defined by speed and direction. From the equation we see that force is directly proportional to both mass and acceleration, which makes sense. To accelerate two objects from rest to the same velocity, you would expect more force to be required to accelerate the more massive object. Likewise, for two objects of the same mass, applying a greater force to one would accelerate it to a greater velocity. Now, let’s rearrange Newton’s second law to solve for acceleration. We get
a = Fnet / m or a = ∑F / m
In this form, we can see that acceleration is directly proportional to force, which we write as
a α Fnet
where the symbol means proportional to. This proportionality mathematically states what we just said in words: acceleration is directly proportional to the net external force. When two variables are directly proportional to each other, then if one variable doubles, the other variable must double. Likewise, if one variable is reduced by half, the other variable must also be reduced by half. In general, when one variable is multiplied by a number, the other variable is also multiplied by the same number. It seems reasonable that the acceleration of a system should be directly proportional to and in the same direction as the net external force acting on the system. An object experiences greater acceleration when acted on by a greater force
It is also clear from the equation a = Fnet / m , that acceleration is inversely proportional to mass, which we write as
a α 1/m
Inversely proportional means that if one variable is multiplied by a number, the other variable must be divided by the same number. Now, it also seems reasonable that acceleration should be inversely proportional to the mass of the system. In other words, the larger the mass (the inertia), the smaller the acceleration produced by a given force. This relationship is illustrated in Figure 4.5, which shows that a given net external force applied to a basketball produces a much greater acceleration than when applied to a car.
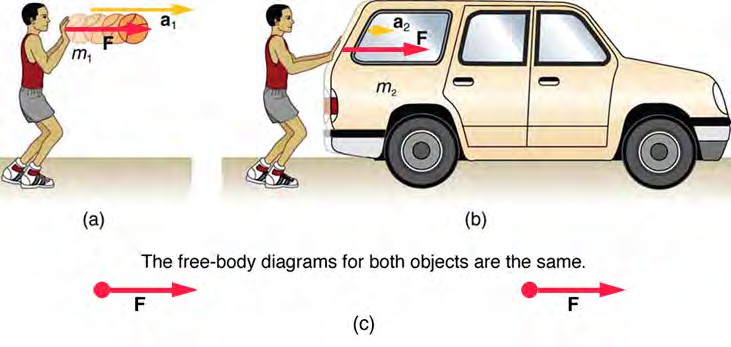
Applying Newton’s Second Law :
Before putting Newton’s second law into action, it is important to consider units. The equation
Fnet = m a is used to define the units of force in terms of the three basic units of mass, length, and time (recall that acceleration has units of length divided by time squared). The SI unit of force is called the newton (abbreviated N) and is the force needed to accelerate a 1-kg system at the rate of 1 m/s 2. That is, because Fnet = m a we have
1 N = 1 Kg X 1 m/s2 = 1 Kg m s -2
One of the most important applications of Newton’s second law is to calculate weight (also known as the gravitational force), which is usually represented mathematically as W. When people talk about gravity, they don’t always realize that it is an acceleration. When an object is dropped, it accelerates toward the center of Earth. Newton’s second law states that the net external force acting on an object is responsible for the acceleration of the object. If air resistance is negligible, the net external force on a falling object is only the gravitational force (i.e., the weight of the object).
Weight can be represented by a vector because it has a direction. Down is defined as the direction in which gravity pulls, so weight is normally considered a downward force. By using Newton’s second law, we can figure out the equation for weight.
Consider an object with mass m falling toward Earth. It experiences only the force of gravity (i.e., the gravitational force or weight), which is represented by W. Newton’s second law states that Because the only force acting on the object is the gravitational force, we have
Fnet = W
We know that the acceleration of an object due to gravity is g, so we have
a = g
Substituting these two expressions into Newton’s second law gives
W = mg
This is the equation for weight—the gravitational force on a mass m. On Earth, g = 9.8 m/s2 so the weight (disregarding for now the direction of the weight) of a 1.0-kg object on Earth is
W = mg = 1 X 9.8 m/s2 = 9.8 N
When the net external force on an object is its weight, we say that it is in freefall. In this case, the only force acting on the object is the force of gravity.
On the surface of Earth, when objects fall downward toward Earth, they are never truly in freefall because there is always some upward force due to air resistance that acts on the object (and there is also the buoyancy force of air, which is similar to the buoyancy force in water that keeps boats afloat).
Gravity varies slightly over the surface of Earth, so the weight of an object depends very slightly on its location on Earth. Weight varies dramatically away from Earth’s surface. On the moon, for example, the acceleration due to gravity is only 1.67 m/s2 . Because weight depends on the force of gravity, a 1.0-kg mass weighs 9.8 N on Earth and only about 1.7 N on the moon.
It is important to remember that weight and mass are very different, although they are closely related. Mass is the quantity of matter (how much stuff) in an object and does not vary, but weight is the gravitational force on an object and is proportional to the force of gravity.
It is easy to confuse the two, because our experience is confined to Earth, and the weight of an object is essentially the same no matter where you are on Earth. Adding to the confusion, the terms mass and weight are often used interchangeably in everyday language; for example, our medical records often show our weight in kilograms, but never in the correct unit of newtons.
Momentum, Impulse, and the Impulse-Momentum Theorem :
Linear momentum is the product of a system’s mass and its velocity. In equation form, linear momentum p is
P = m V
You can see from the equation that momentum is directly proportional to the object’s mass (m) and velocity (v). Therefore, the greater an object’s mass or the greater its velocity, the greater its momentum.
A large, fast-moving object has greater momentum than a smaller, slower object. Momentum is a vector and has the same direction as velocity v. Since mass is a scalar, when velocity is in a negative direction (i.e., opposite the direction of motion), the momentum will also be in a negative direction; and when velocity is in a positive direction, momentum will likewise be in a positive direction. The SI unit for momentum is kg m/s.
Momentum is so important for understanding motion that it was called the quantity of motion by physicists such as Newton. Force influences momentum, and we can rearrange Newton’s second law of motion to show the relationship between force and momentum.
Newton actually stated his second law of motion in terms of momentum: The net external force equals the change in momentum of a system divided by the time over which it changes. The change in momentum is the difference between the final and initial values of momentum. In equation form, this law is
Fnet = ΔP / Δt
where Fnet is the net external force, ΔP is the change in momentum, and Δt is the change in time. We can solve for ΔP by rearranging the equation
ΔP = Fnet Δt
Fnet Δt is known as impulse and this equation is known as the impulse-momentum theorem.
From the equation, we see that the impulse equals the average net external force multiplied by the time this force acts. It is equal to the change in momentum. The effect of a force on an object depends on how long it acts, as well as the strength of the force. Impulse is a useful concept because it quantifies the effect of a force. A very large force acting for a short time can have a great effect on the momentum of an object, such as the force of a racket hitting a tennis ball. A small force could cause the same change in momentum, but it would have to act for a much longer time
Newton’s Second Law in Terms of Momentum
When Newton’s second law is expressed in terms of momentum, it can be used for solving problems where mass varies, since ΔP = Δ(mv) . In the more traditional form of the law that you are used to working with, mass is assumed to be constant. In fact, this traditional form is a special case of the law, where mass is constant.Fnet = ma is actually derived from the equation:
Fnet = ΔP / Δt
The change in momentum ΔP is given by
ΔP = Δ (mv)
If the mass of the system is constant, then
Δ (mv) = m Δv
By substituting m Δv for ΔP , Newton’s second law of motion becomes
Fnet = ΔP / Δt
= m Δv / Δt for a constant mass
Since Δv / Δt = a , we get
Fnet = ma , when the mass of the system is constant
Conservation of Momentum
It is important we realize that momentum is conserved during collisions, explosions, and other events involving objects in motion. To say that a quantity is conserved means that it is constant throughout the event. In the case of conservation of momentum, the total momentum in the system remains the same before and after the collision.
You may have noticed that momentum was not conserved , where forces acting on the objects produced large changes in momentum. Why is this? The systems of interest considered in those problems were not inclusive enough. If the systems were expanded to include more objects, then momentum would in fact be conserved in those sample problems. It is always possible to find a larger system where momentum is conserved, even though momentum changes for individual objects within the system. For example, if a football player runs into the goalpost in the end zone, a force will cause him to bounce backward. His momentum is obviously greatly changed, and considering only the football player, we would find that momentum is not conserved. However, the system can be expanded to contain the entire Earth. Surprisingly, Earth also recoils—conserving momentum—because of the force applied to it through the goalpost. The effect on Earth is not noticeable because it is so much more massive than the player, but the effect is real. Next, consider what happens if the masses of two colliding objects are more similar than the masses of a football player and Earth—in the example shown in Figure 8.4 of one car bumping into another. Both cars are coasting in the same direction when the lead car, labeled m2 , is bumped by the trailing car, labeled m1 . The only unbalanced force on each car is the force of the collision, assuming that the effects due to friction are negligible. Car m1 slows down as a result of the collision, losing some momentum, while car m2 speeds up and gains some momentum. If we choose the system to include both cars and assume that friction is negligible, then the momentum of the two-car system should remain constant. Now we will prove that the total momentum of the two-car system does in fact remain constant, and is therefore conserved
Newton’s Third Law of Motion :
Newton’s third law of motion states that whenever a first object exerts a force on a second object, the first object experiences a force equal in magnitude but opposite in direction to the force that it exerts.
Newton’s third law of motion tells us that forces always occur in pairs, and one object cannot exert a force on another without experiencing the same strength force in return. We sometimes refer to these force pairs as action-reaction pairs, where the force exerted is the action, and the force experienced in return is the reaction (although which is which depends on your point of view).
Newton’s third law is useful for figuring out which forces are external to a system. Recall that identifying external forces is important when setting up a problem, because the external forces must be added together to find the net force.
We can see Newton’s third law at work by looking at how people move about. Consider a swimmer pushing off from the side of a pool. He pushes against the pool wall with his feet and accelerates in the direction opposite to the push. The wall has thus exerted on the swimmer a force of equal magnitude but in the direction opposite that of his push. You might think that two forces of equal magnitude but that act in opposite directions would cancel, but they do not because they act on different systems.
Similarly, a car accelerates because the ground pushes forward on the car’s wheels in reaction to the car’s wheels pushing backward on the ground. You can see evidence of the wheels pushing backward when tires spin on a gravel road and throw rocks backward.
Birds fly by exerting force on air in the direction opposite that in which they wish to fly. For example, the wings of a bird force air downward and backward in order to get lift and move forward
Applying Newton’s Third Law :
The gravitational force (or weight) acts on objects at all times and everywhere on Earth. We know from Newton’s second law that a net force produces an acceleration; so, why is everything not in a constant state of freefall toward the center of Earth? The answer is the normal force. The normal force is the force that a surface applies to an object to support the weight of that object; it acts perpendicular to the surface upon which the object rests. If an object on a flat surface is not accelerating, the net external force is zero, and the normal force has the same magnitude as the weight of the system but acts in the opposite direction. In equation form, we write that
N = mg
Note that this equation is only true for a horizontal surface.
The word tension comes from the Latin word meaning to stretch. Tension is the force along the length of a flexible connector, such as a string, rope, chain, or cable. Regardless of the type of connector attached to the object of interest, one must remember that the connector can only pull (or exert tension) in the direction parallel to its length. Tension is a pull that acts parallel to the connector, and that acts in opposite directions at the two ends of the connector. This is possible because a flexible connector is simply a long series of action-reaction forces, except at the two ends where outside objects provide one member of the actionreaction forces.
Consider a person holding a mass on a rope, as shown in below Figure
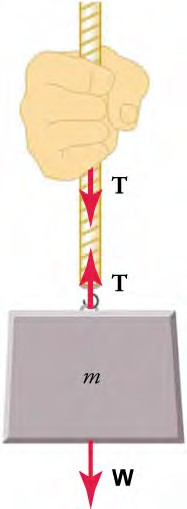
Tension in the rope must equal the weight of the supported mass, as we can prove by using Newton’s second law. If the 5 kg mass in the figure is stationary, then its acceleration is zero, so Fnet = 0 . The only external forces acting on the mass are its weight W and the tension T supplied by the rope. Summing the external forces to find the net force, we obtain
Fnet = T – W = 0
where T and W are the magnitudes of the tension and weight, respectively, and their signs indicate direction, with up being positive. By substituting mg for Fnet and rearranging the equation, the tension equals the weight of the supported mass, we get
T = W = mg
For a 5 Kg mass , the we see that
T = mg = 5 Kg X 9.8 m/s2 = 49 N
Another example of Newton’s third law in action is thrust. Rockets move forward by expelling gas backward at a high velocity. This means that the rocket exerts a large force backward on the gas in the rocket combustion chamber, and the gas, in turn, exerts a large force forward on the rocket in response. This reaction force is called thrust.
Take Quiz
1. An object is at rest. Two forces, X and Y, are acting on it. Force X has a magnitude of x and acts in the downward direction. What is the magnitude and direction of Y?
a. The magnitude is x and points in the upward direction.
b. The magnitude is 2x and points in the upward direction.
c. The magnitude is x and points in the downward direction.
d. The magnitude is 2x and points in the downward direction
ANSWER
a. The magnitude is x and points in the upward direction.
2) Three forces, A, B, and C, are acting on the same object with magnitudes a, b, and c, respectively. Force A acts to the right, force B acts to the left, and force C acts downward. What is a necessary condition for the object to move straight down?
a. The magnitude of force A must be greater than the magnitude of force B, so a > b
b. The magnitude of force A must be equal to the magnitude of force B, so a = b
c. The magnitude of force A must be greater than the magnitude of force C, so A > C.
d. The magnitude of force C must be greater than the magnitude of forces A or B, so A < C > B
ANSWER
d. The magnitude of force C must be greater than the magnitude of forces A or B, so A < C > B
3. Two people push a cart on a horizontal surface by applying forces F1 and F2 in the same direction. Is the magnitude of the net force acting on the cart, Fnet , equal to, greater than, or less than F1 + F2 ? Why?
a. Fnet < F1 + F2 because the net force will not include the frictional force.
b. Fnet = F1 + F2 because the net force will not include the frictional force
c. Fnet < F1 + F2 because the net force will include the component of frictional force
d. Fnet = F1 + F2 because the net force will include the frictional force
ANSWER
b. Fnet = F1 + F2 because the net force will not include the frictional force
4) True or False: A book placed on a balance scale is balanced by a standard 1-kg iron weight placed on the opposite side of the balance. If these objects are taken to the moon and a similar exercise is performed, the balance is still level because gravity is uniform on the moon’s surface as it is on Earth’s surface.
a. True
b. False
ANSWER
b. False
5) A car weighs 2,000 kg. It moves along a road by applying a force on the road with a parallel component of 560 N. There are two passengers in the car, each weighing 55 kg. If the magnitude of the force of friction experienced by the car is 45 N, what is the acceleration of the car?
a. 0.244 m/s2
b. 0.265 m/s2
c. 4.00 m/s2
d. 4.10 m/s2
ANSWER
a. 0.244 m/s2
6. A person pushes an object of mass 5.0 kg along the floor by applying a force. If the object experiences a friction force of 10 N and accelerates at 18 m/s2 , what is the magnitude of the force exerted by the person?
a. −90 N
b. −80 N
c. 90 N
d. 100 N
ANSWER
d. 100 N
7. A 2,000-kg car is sitting at rest in a parking lot. A bike and rider with a total mass of 60 kg are traveling along a road at 10 km/h. Which system has more inertia? Why?
a. The car has more inertia, as its mass is greater than the mass of the bike.
b. The bike has more inertia, as its mass is greater than the mass of the car.
c. The car has more inertia, as its mass is less than the mass of the bike.
d. The bike has more inertia, as its mass is less than the mass of the car
ANSWER
a. The car has more inertia, as its mass is greater than the mass of the bike.
8. Two people push a 2,000-kg car to get it started. An acceleration of at least 5.0 m/s2 is required to start the car. Assuming both people apply the same magnitude force, how much force will each need to apply if friction between the car and the road is 300 N?
a. 4850 N
b. 5150 N
c. 97000 N
d. 10300 N
ANSWER
b. 5150 N
9. A 55-kg lady stands on a bathroom scale inside an elevator. The scale reads 70 kg. What do you know about the motion of the elevator?
a. The elevator must be accelerating upward.
b. The elevator must be accelerating downward.
c. The elevator must be moving upward with a constant velocity.
d. The elevator must be moving downward with a constant velocity.
ANSWER
a. The elevator must be accelerating upward.
10. Are rockets more efficient in Earth’s atmosphere or in outer space? Why?
a. Rockets are more efficient in Earth’s atmosphere than in outer space because the air in Earth’s atmosphere helps to provide thrust for the rocket, and Earth has more air friction than outer space.
b. Rockets are more efficient in Earth’s atmosphere than in outer space because the air in Earth’s atmosphere helps to provide thrust to the rocket, and Earth has less air friction than the outer space.
c. Rockets are more efficient in outer space than in Earth’s atmosphere because the air in Earth’s atmosphere does not provide thrust but does create more air friction than in outer space.
d. Rockets are more efficient in outer space than in Earth’s atmosphere because the air in Earth’s atmosphere does not provide thrust but does create less air friction than in outer space.
ANSWER
c. Rockets are more efficient in outer space than in Earth’s atmosphere because the air in Earth’s atmosphere does not provide thrust but does create more air friction than in outer space.